Soil erosion and deposition modeling
Introduction
Steve Warren, Helena Mitasova
Military training activities can have adverse consequences on natural resources. Tracked and wheeled vehicles compact the soil and damage or destroy both herbaceous and woody vegetation (Wilson 1988, Shaw and Diersing 1990, Thurow et al. 1993). Exploding munitions can cause soil displacement, wildfire, and death or injury to plants across large areas. Construction of emplacements, anti-tank ditches and fox-holes cause extensive localized damage. Even bivouac sites can result in soil compaction, loss of herbaceous plant cover and damage to trees (Trumball et al. 1994). Soil erosion may accelerate as the soil surface becomes increasingly disturbed and protective vegetation is lost as a result of the cumulative impacts of military training. If allowed to continue unchecked, extensive damage from gullying may occur. Such damage is not only expensive to repair, but also diminishes the realism and quality of the military training experience, and jeopardizes the safety of soldiers and equipment.
Soil erosion on military training facilities may also create off-site impacts. Nationally, sediment is the single largest contributor to non-point source runoff pollution. As much as 92% of suspended sediments in waterways is attributable to non-point sources (Clark et al. 1985). Section 208 of the federal Water Pollution Control Act and section 319 of the 1987 amendment to the federal Clean Water Act require resource managers and planners to assess the extent of non-point source water pollution problems and to develop and implement area-wide best management practices (BMPs) to prevent water pollution from non-point sources.
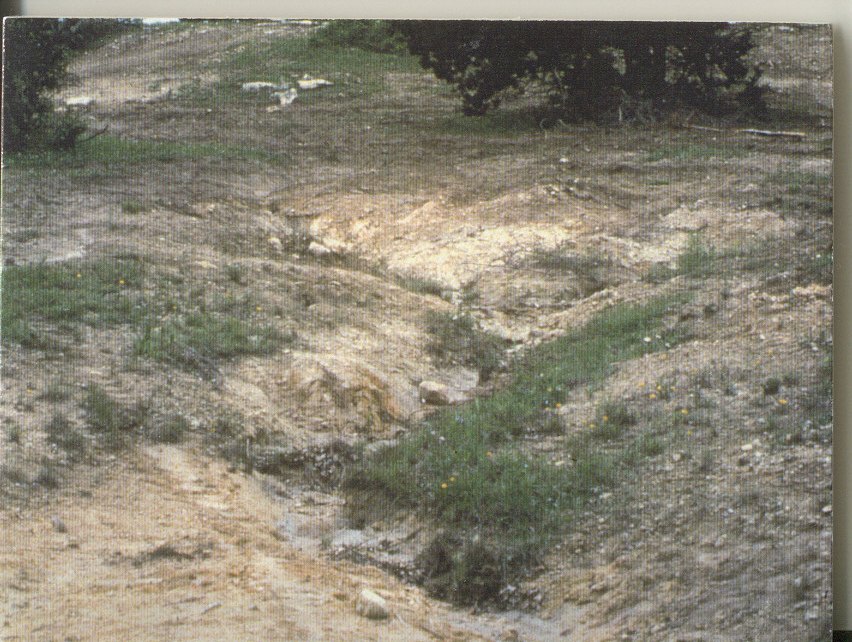
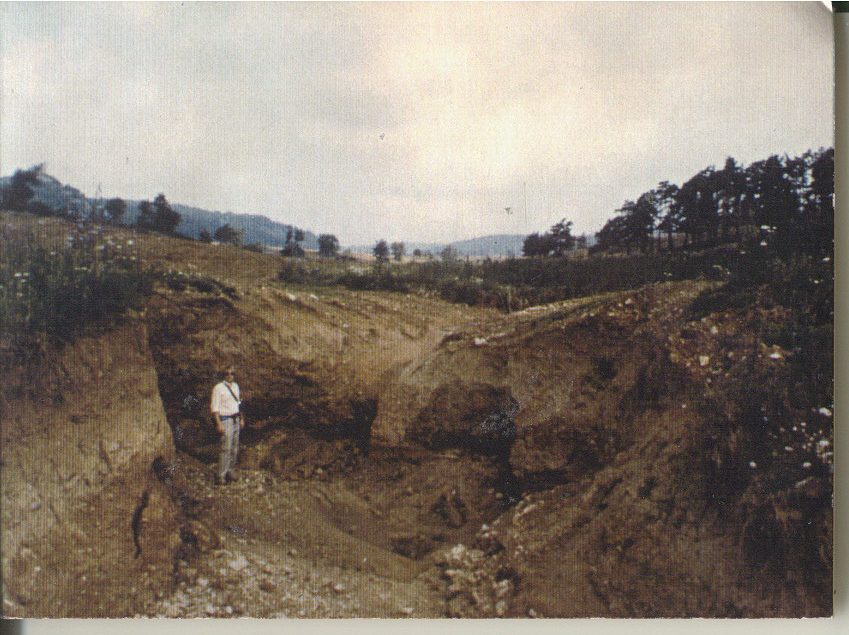
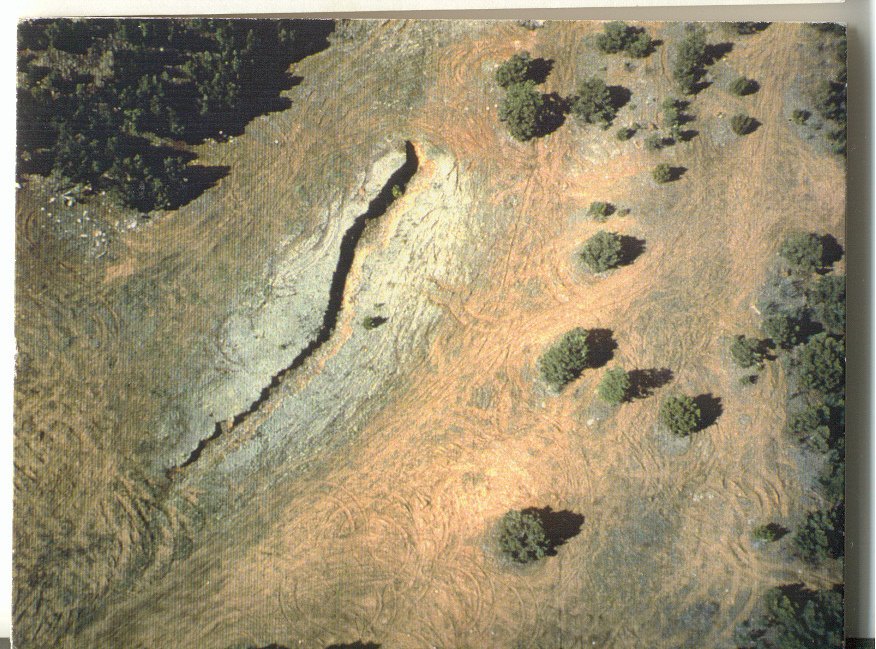
According to Department of Defense Instruction 4715, Enclosure 3, Section 4.b.4, dated 18 March 2011, the “DoD shall prevent and control soil erosion, and implement soil conservation measures…” in accordance with United States Code, Title 16, Chapter 3B, Soil Conservation. Measuring soil erosion and resultant sediment deposition in order to determine when an installation is out of compliance is difficult and subject to considerable error and subjectivity. 7 CFR 610.12 prescribes the use of the Universal Soil Loss Equation (USLE; Wischmeier and Smith 1978) and the Revised Universal Soil Loss Equation (RUSLE; Renard et al. 1997) for measuring soil erosion by the Natural Resources Conservation Service (NRCS). At least part of popularity of these models can be attributed to the ease with which they are applied. However, a major drawback of these and even many of the more complex new-generation process-based models (e.g., Water Erosion Prediction Project [WEPP]; Flanagan and Nearing 1995) is the 1-dimensional approach used to account for the effects of topography. Landscapes have generally been treated as homogenous, planar features. Average erosion rates have been assigned to entire hillslopes and watersheds, thus providing no information regarding sources and sinks of eroded materials. Neither approach provides adequate spatially distributed information on erosion necessary to effectively optimize erosion and sediment control efforts.
A second shortcoming of the USLE and the RUSLE is that they predict soil erosion only; they do not predict sediment deposition. Furthermore, both models predict erosion ‘universally’, even where deposition may occur. Thus, at landscape or watershed scales, the spatial distribution of soil erosion as predicted by these models will likely misrepresent actual conditions and will tend to overestimate erosion (e.g., Jensen 1983, Busacca et al. 1993). The only practical way to apply the models is to identify a priori those portions of the landscape subject to deposition and exclude them from analysis (Mitasova et al. 1997).